Designing the Best Possible Solar Cell, by MacGyvering a Bit of Quantum Supremacy
January 26, 2021
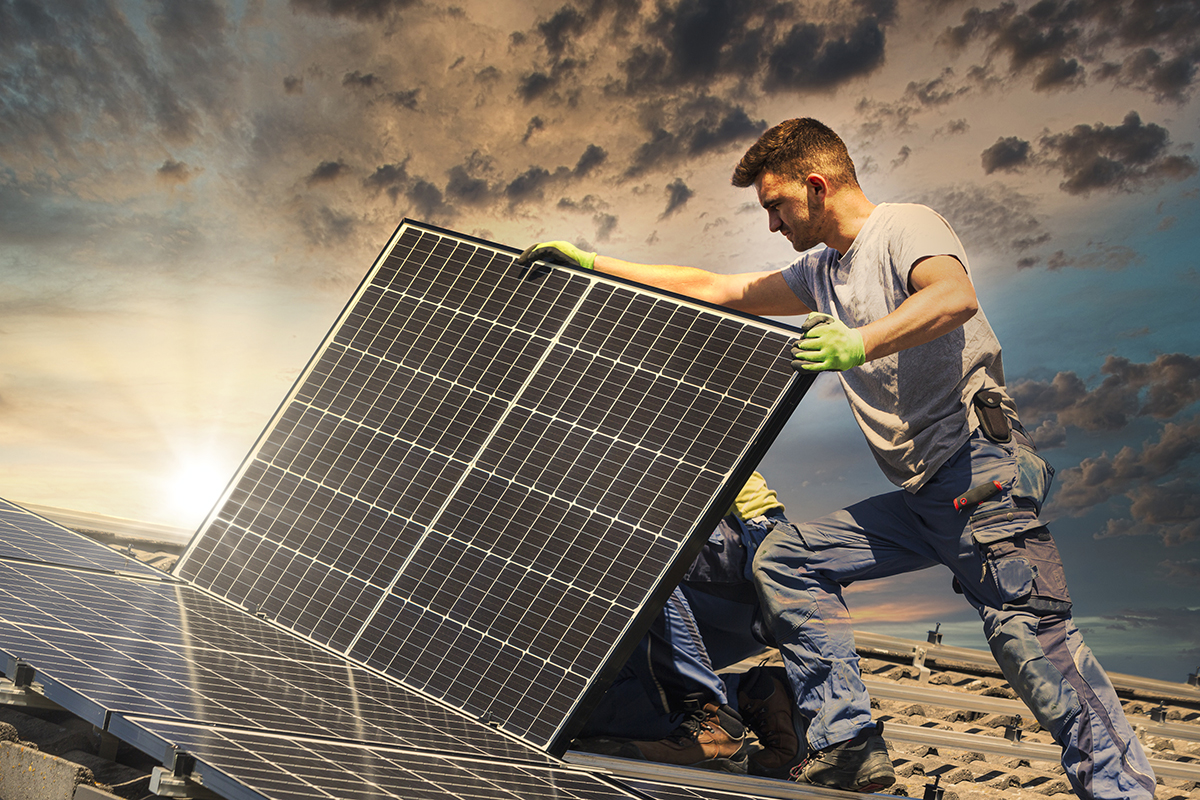
While solar represents 15% of all renewable electricity generation today (compared to wind power at 38% and water power at 37%) solar is expected to surpass both and account for almost half of all electricity from renewable sources in 2050, according to the U.S. Energy Information Administration. Considering that total renewable electricity generation is expected to triple by then, the total amount of solar power would increase almost ten-fold. Instead of installing ten times more solar panels, which are made with fairly expensive materials, it would be more affordable to install fewer and more efficient solar panels—that’s what LSU researchers are working on now, thanks to a five-year grant from the U.S. Department of Energy.
LSU physicists and material scientists are building a quantum simulator with a gold chip to discover better materials for solar cells. Ideal materials must introduce some amount of mayhem, the researchers say, to get more light to go through.
Light consists of photons, and they move in mysterious ways. Because photons are what physicists call “discrete particles,” and thus subject to quantum effects, they don’t abide by what we’d consider normal rules. Traveling from the sun, photons from sunlight have a mindboggling number of options open to them. Indeed, it’s difficult to predict where even a single photon will go since it can be in two places at the same time (a quantum effect called superposition). Superposition is why that relatively cute cat that belonged to a man named Schrödinger (or in truth, probably to his grant-aunt) could be described as simultaneously dead and alive. And to put this quantum lawlessness into yet another context, you could consider Newton’s notorious-yet-unconfirmed incident under the apple tree—if the apple that supposedly fell and hit him on the head (helping him discover gravity) had been a photon instead, it might have struck a nearby church bell while also serving as a delightful snack for Newton’s horse.
“Sunlight is chaotic, crazy light,” said Omar Magaña-Loaiza, assistant professor and head of the quantum photonics lab in the LSU Department of Physics & Astronomy. “Sunlight will be transported differently from other kinds of light, and this is a major challenge in the design of solar cells.”
“ A photon that becomes lodged inside a material usually gets absorbed—nothing comes out. ”
Most solar cells on the market today contain a crystalline silicon to help transport photons in the conversion of light energy into electricity. But even on a sunny day, the harvest can be uneven. Apart from being unpredictable, photons are subject to so-called localization effects due to interferences on the atomic level. Localization can cause photons to get “stuck” in seemingly random patterns and cluster in odd places. In solar cells, this is a big problem. A photon that becomes lodged inside a material usually gets absorbed—nothing comes out. What the LSU researchers are trying to do is break localization effects to make solar cells more efficient. With a recent grant from the U.S. Department of Energy, they are building a quantum simulator to study the transport of light and are now figuring out how to manipulate it in just the right way to be able to harvest more photons faster.
“Materials are basically networks,” Magaña-Loaiza said. “Networks of atoms. And if you order the atoms in a certain way you can slow down or speed up the photons as they travel through the material, and with just the right amount of disorder in the network, you can break the localization effects.”
By changing different parameters in their simulator, Magaña-Loaiza and You can mimic the transport of light in multiple crystalline materials that could be used to fabricate solar cells. Their goal is to be able to tell the materials scientists what materials to make—which properties would be required.
“In a nice and strong material, such as a well-organized and well-behaved crystal, you can get localization,” Magaña-Loaiza explained. “So, we have to add randomness in a clever way to boost the transport. Just the right kind of disturbance can get the photons from the entrance to the exit. The material cannot allow certain interference conditions, and we’re figuring out which ones.”
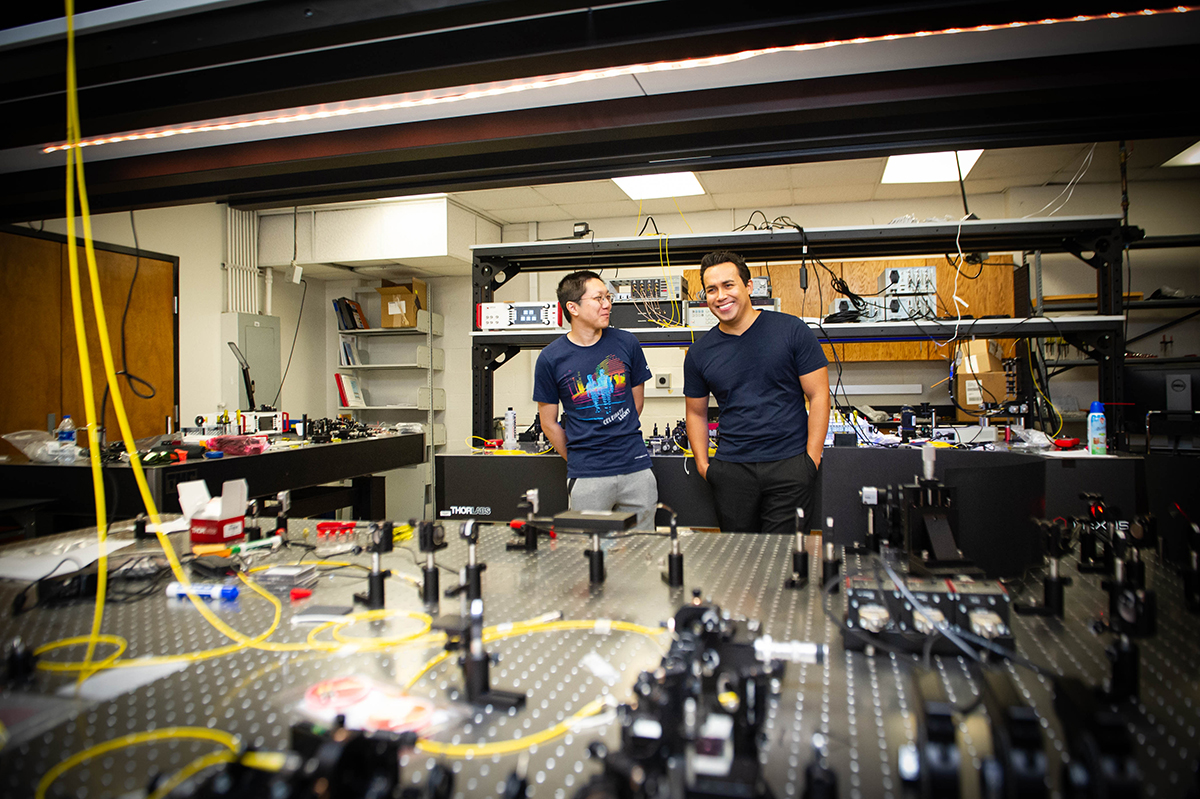
Chenglong You and Omar Magaña-Loaiza in the LSU Quantum Photonics Lab.
– Elsa Hahne
If you’ve ever been to an IKEA, you might grasp how this transport works (or doesn’t). A bright yellow line on the floor marks a winding path through the store, leading customers past every single display. Naturally, customers tend to cluster in certain areas, such as near the bookcases and home organization items. But if you were to hire a few scary characters and place them next to the Billy, Råskog, and Rast, you’d likely get movement and more people out the door. This is the kind of controlled mayhem Magaña-Loaiza and his colleague Chenglong You, an LSU postdoctoral researcher, are trying to stage. They’re not making or testing materials or solar cells per se. They’re “simply” simulating the optimal conditions for light transport using a custom-built quantum device that’s not unlike a fancy calculator.
Because of the unfathomable number of possibilities for how photons can move through space, you could not run this kind of simulation on a regular computer—not even a supercomputer. A quantum leap is required. While the late LSU physicist Jon Dowling, a world-renowned expert in quantum information science, addressed many of these transport problems in quantum random walk networks, Magaña-Loaiza and You are building a physical device to mimic the “chaotic, crazy” paths that not just individual photons but multiple photons can take. And to simulate more options more quickly, they’ve built their apparatus around a sliver of gold with slits cut at precise intervals (manufactured at the LSU Shared Instrumentation Facility, or SIF) to upgrade the capacity of their calculator by working with not just photons but plasmons—electromagnetic waves around the surfaces of metals, such as gold. Photons can excite plasmons, which move in even more mysterious ways. To understand what plasmons add to Magaña-Loaiza’s and You’s research, you could imagine a highway toll booth with an inquisitive attendant. The attendant asks everyone who passes through about their final destination, but instead of manning a single-passenger lane, the attendant works the HOV lane. For every vehicle that passes through, the attendant learns the final destination of several passengers at once—multiple drop-off points. The vans and buses are the plasmons, while Magaña-Loaiza and You take turns as the attendant.
“ Because of the unfathomable number of possibilities for how photons can move through space, you could not run this kind of simulation on a regular computer—not even a supercomputer. A quantum leap is required. ”
“Including plasmons in our work allows more options for where the light can go,” Magaña-Loaiza said. “We use plasmons to simulate complex physical effects and this more sophisticated system allows us to handle all possibilities, especially since plasmons are ‘lossy’ fields and we then can study the losses and try to use them in our favor. You can’t avoid losses; you have to live with them. So, working with plasmons helps us understand and utilize the loss instead of trying to avoid its adversary effects—that’s a particular thing for our platform.”
Plasmons help the researchers mimic more disorder. Typically, you wouldn’t want disorder in a material or in a lab, but in this case, Magaña-Loaiza and You need disorder to simulate some of the crazy things that happen with light.
“Lots of companies are trying to work on improving the efficiency of solar cells, but it’s very difficult because it is challenging to simulate the underlying physics that define light transport,” Magaña-Loaiza continued. “Photons don’t necessarily move in ordered patterns, like straight through a crystal. Instead, they can loop around and induce complex superpositions.”
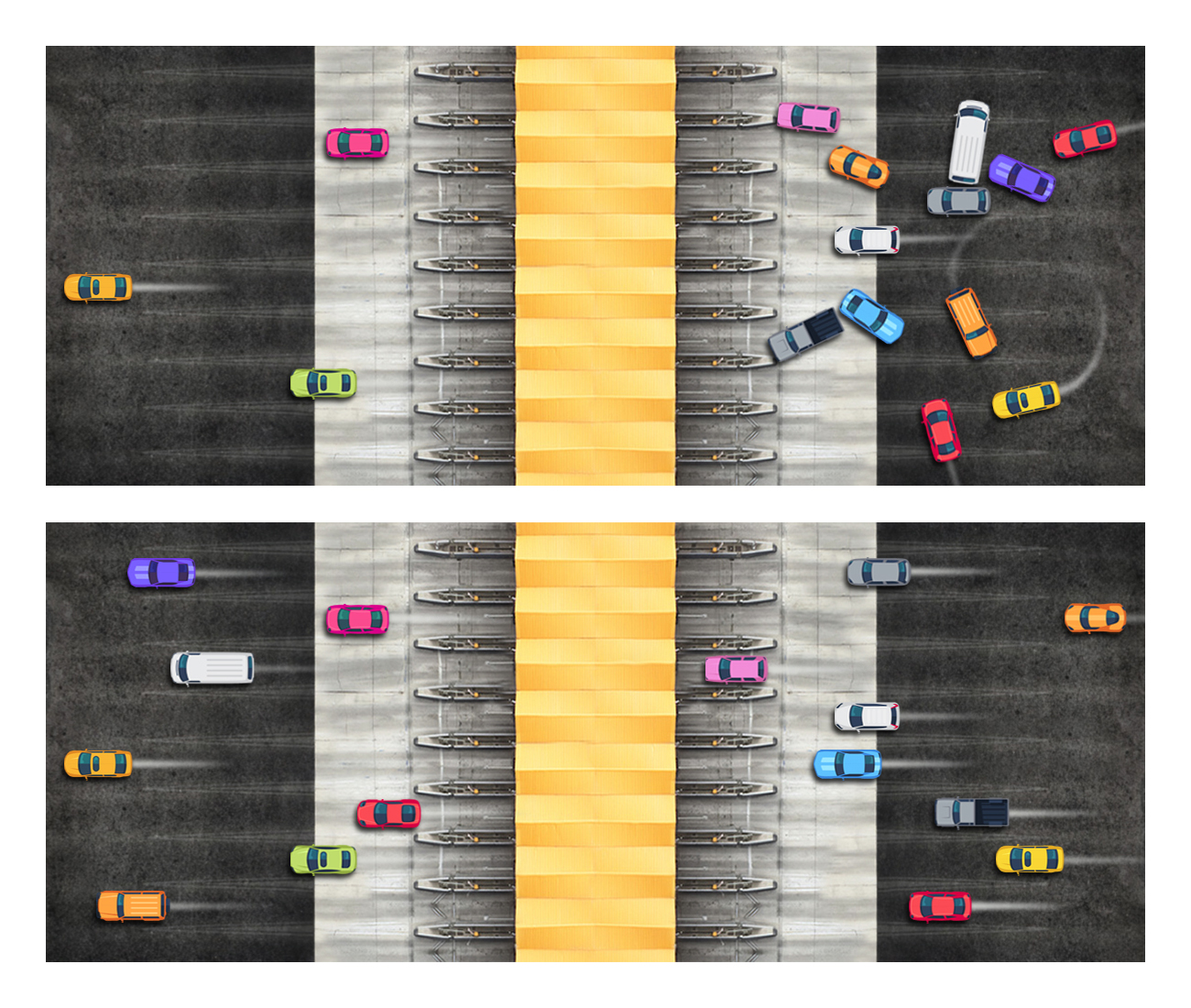
Before/after: Photons, because they’re quantized and therefore subject to quantum effects, can behave like unruly, lawless drivers. If you think of today’s solar cells as if they’re highway toll booths, the drivers would be driving in circles, backing up and going sideways, and generally holding up traffic instead of all traveling through at good speed. This is why it’s so difficult to turn light energy into electrical energy in solar cells at steady and predictable rates—even when the sun is shining all day. The reason for this mayhem is something physicists know as “localization effects.” What LSU researchers Omar Magaña-Loaiza and Chenglong You are trying to do is to break the localization effects by designing better solar cell materials that will allow more photons to pass through faster and thus increase solar cell efficiency.
– Elsa Hahne
While “quantum supremacy” has been tossed around quite a bit lately after Google claimed achieving it in 2019, Magaña-Loaiza is someone lukewarm on the term, which simply means that you’ve been able to do something with a quantum device that you couldn’t do without it. This holds true for Magaña-Loaiza’s and You’s simulator.
“Jon Dowling performed research devoted to the development of photonic circuits for information processing and identified specific conditions that cannot be simulated on a classical computer,” said Magaña-Loaiza about the late LSU scientist who encouraged him to join his team at LSU. “He also identified specific multiparticle events that have enormous potential for quantum computers. I was blown away when I read his work in grad school. Now, our solution, a quantum-enhanced simulator with a chip, is like a specialized quantum computer—we will use it to help solve the particular problem our simulator was built to solve.”
Magaña-Loaiza points out that it would be possible to build similar quantum simulators for other specific tasks, such as how to design drugs or predict traffic jams.
“Once we’re done with this project, we’ll be able to say, ‘Use this design to build your solar cell and localization won’t happen,’ or, ‘Make this change to speed up the photons as they go through,’” he said. “But you could build a similar setup to help calculate answers to a lot of different questions.”
“We’re merging two research fields together here,” Magaña-Loaiza continued. “Quantum optics, which is what we normally work on, and plasmonics, which belongs more in the classical, non-quantum, realm. We’re not experts on materials and we’re not making crystals or building solar cells—we’re simply building and using our platform to simulate something that cannot be simulated on regular computers. We’re simulating what otherwise cannot be simulated, but our platform is very complex—it has to be. You can’t just put some light in there and click ‘play.’ We have to study one parameter at a time, and we’re doing this with a single photon as well as entangled multiphoton packets where we can control the interaction. We can’t simulate the whole thing at once, but we can do it in pieces and figure out how much of the light is reflected, transmitted, and absorbed.”
”LSU’s exciting research on materials and light for improved solar cells could have positive implications for some of our customers’ resilience and efficiency efforts.”
Stephen Toups, Turner Industries
Baton Rouge-based Turner Industries is a global company that provides turnkey services and solutions for the heavy industrial sector, supporting clients who produce fuel, energy, and essential products. The company’s president, Stephen Toups, is interested in the frontier work on solar cells the LSU quantum photonics group is doing; he understands that meeting demand for a growing world population will require an array of efficient energy sources.
“As one of the nation’s leading heavy industrial contractors, our company is committed to championing innovative solutions to help our customers secure and sustain operations in often challenging environments,” Toups said. “LSU’s exciting research on materials and light for improved solar cells could have positive implications for some of our customers’ resilience and efficiency efforts.”